티스토리 뷰
Part 3 Neurophysiology of Hand Function
Mechanoreceptors: Glabrous Skin
08/09/2024
How do you categorize types of Mechanoreceptors?
My summary: FA I, FA II, SA I, SA II
Fast-adapting: sensitive to movements
Slow-adapting: sensitive to static stimulus
type I: Fine-spatial sensing
type II: Large-spatial sensing
First summary of ChatGPT
The categorization of afferent units into Fast-Adapting (FA) and Slow-Adapting (SA) is based on how they respond to sustained stimuli and their ability to detect changes in tactile input over time.
Fast-Adapting (FA) Units:
- Response to Stimuli: FA units respond quickly to the onset and offset of a stimulus but do not maintain a response if the stimulus is constant. They are highly sensitive to changes in stimulation, such as vibrations or sudden movements, but their activity diminishes rapidly when the stimulus is steady.
- Function: FA units are primarily involved in detecting dynamic tactile events, like the initial contact with an object, slip, or vibrations. They are essential for perceiving textures, detecting motion, and providing feedback during tasks like gripping.
- Examples:
- FA I units: Detect low-frequency vibrations and textures.
- FA II units: Detect high-frequency vibrations and transient events.
Slow-Adapting (SA) Units:
- Response to Stimuli: SA units continue to respond as long as the stimulus is present. They fire at a steady rate during sustained pressure or stretch, making them suited for detecting static features of an object, such as shape, edges, and skin stretch.
- Function: SA units are crucial for perceiving the size, shape, and pressure of objects, as well as for maintaining an awareness of hand and finger positions over time.
- Examples:
- SA I units: Detect fine spatial details like edges and textures.
- SA II units: Respond to skin stretch and are important for sensing hand posture and object shape.
Second summary of ChatGPT with examples
In human skin, there are four main types of mechanoreceptive afferent units: FA I, SA I, FA II, and SA II. Each of these units is associated with specific sensory functions, related to their unique structures and the density at which they are distributed across the palm. Here's a summary of each:
1. FA I (Fast-Adapting Type I)
- Role: FA I units are specialized in detecting dynamic, low-frequency vibrations and are crucial for sensing texture and small movements between the skin and an object, such as when you feel a textured surface with your fingertips.
- Example: When you grip an object and it starts to slip slightly, FA I units detect this micro-slip and help you adjust your grip.
- Density: They are densely distributed, especially in the fingertips (about 1.5 units/mm²).
- Receptive Fields: FA I units have small receptive fields (about 3-5 mm in diameter), allowing them to detect fine details of textures.
2. SA I (Slow-Adapting Type I)
- Role: SA I units respond to static stimuli and are sensitive to edges, shapes, and fine details, playing a key role in perceiving object form and surface texture.
- Example: When you run your fingers over a piece of cloth and can feel the fabric's weave, SA I units are responsible for detecting these fine spatial details.
- Density: They have a relatively high density (about 1 unit/mm²).
- Receptive Fields: SA I units have very small receptive fields (2-3 mm in diameter), which enable precise spatial resolution of fine textures.
3. FA II (Fast-Adapting Type II)
- Role: FA II units are specialized in detecting high-frequency vibrations and transient events, like an object making brief contact with the skin.
- Example: When you use a tool and can feel vibrations traveling through it, FA II units are detecting those vibrations.
- Density: These units are less densely packed (around 350 units per finger), with fewer units spread across the palm.
- Receptive Fields: FA II units have large receptive fields, meaning they are not as effective in detecting fine spatial details but are excellent for detecting vibrations over larger areas.
4. SA II (Slow-Adapting Type II)
- Role: SA II units respond to skin stretch and are important for perceiving the shape of large objects and understanding the position and movement of fingers and hands.
- Example: When holding a large object, SA II units help you perceive its overall shape and your hand's position around it.
- Density: SA II units are relatively sparsely distributed compared to SA I and FA I.
- Receptive Fields: SA II units have large receptive fields, which are less suited to detecting fine details but are essential for understanding larger-scale deformations and hand posture.
Density and Receptive Fields Relation to Roles
- FA I and SA I: These units have high densities and small receptive fields, making them crucial for tasks requiring detailed spatial information, like texture discrimination and edge detection.
- FA II and SA II: These units have lower densities and larger receptive fields, aligning them with functions that involve detecting broader, less detailed stimuli, such as vibrations and skin stretch over larger areas.
1. FA I (Fast-Adapting Type I)
- Example: When you grip an object and it starts to slip slightly, FA I units detect this micro-slip and help you adjust your grip.
2. SA I (Slow-Adapting Type I)
- Example: When you run your fingers over a piece of cloth and can feel the fabric's weave, SA I units are responsible for detecting these fine spatial details.
3. FA II (Fast-Adapting Type II)
- Example: When you use a tool and can feel vibrations traveling through it, FA II units are detecting those vibrations.
4. SA II (Slow-Adapting Type II)
- Example: When holding a large object, SA II units help you perceive its overall shape and your hand's position around it.
What are RA, PC, NP I, II, and III?
The terms RA, PC, NP I, II, and III refer to specific types of mechanoreceptors or afferent units, primarily in the context of tactile sensation research in humans and animals, particularly monkeys. Here’s what each term means:
- RA (FA I): Detect low-frequency vibrations, essential for texture perception.
- PC (FA II): Detect high-frequency vibrations, crucial for sensing fine vibrations and remote events.
- NP I (SA I): Sensitive to static pressure and fine spatial details, important for texture and shape perception.
- NP II (FA I): Similar to RA units, involved in detecting flutter and initial contact.
- NP III (SA II): Sensitive to skin stretch, involved in detecting hand posture and object shape.
RA (Rapidly Adapting), PC (Pacinian Corpuscles), NP I, II, and III (Non-Pacinian I, II, III)
What are the main types of mechanoreceptors in hairy skin (dorsal skin)?
- Slowly Adapting Mechanoreceptors: Merkel cells (fine details, texture) and Ruffini endings (skin stretch, object shape).
- Rapidly Adapting Mechanoreceptors: Hair-follicle receptors (hair movement, light touch), field units (pressure changes, multiple points of contact), and Pacinian corpuscles (vibrations, fine tactile details).
- Unmyelinated Tactile Units: Possibly involved in emotional aspects of touch.
These mechanoreceptors collectively allow hairy skin to detect a range of tactile stimuli, from fine textures and vibrations to light touches and skin stretch, contributing to both precise and affective touch sensations.
What are the categories of Meissner corpuscles, Merkel cells, Pacinian corpuscles and Ruffini endings in RA I, II and SA I, II?
Meissner corpuscles: RA I (Rapidly Adapting Type I)
These are located close to the surface of the skin and detect light touch and vibration.
Merkel cells: SA I (Slowly Adapting Type I)
Merkel cells are also found near the skin surface and are responsible for sensing pressure and texture.
Pacinian corpuscles: RA II (Rapidly Adapting Type II)
Located deeper in the skin, they detect high-frequency vibrations and deep pressure.
Ruffini endings: SA II (Slowly Adapting Type II)
These are found in deeper tissues and respond to skin stretch and sustained pressure
What are the mechanorecptors in muscles? What do they do?
In muscles, three main types of mechanoreceptors provide crucial information to the central nervous system (CNS) about muscle length and force. These mechanoreceptors play a vital role in proprioception, which is the body's ability to sense its position and movement in space. Here's a summary of these mechanoreceptors:
1. Primary and Secondary Muscle Spindle Receptors
- Location: Muscle spindles, which are elongated structures within the muscle.
- Function: These are stretch receptors that detect changes in muscle length. They provide the CNS with information about muscle stretch and limb position.
- Primary Spindle Receptors:
- Afferent Fibers: Innervated by Group Ia (large myelinated) afferent fibers.
- Sensitivity: Highly sensitive to the velocity of muscle stretch and respond vigorously to rapid changes. They are nonlinear in their response and are influenced by factors like muscle length, velocity of stretch, and fusimotor system activity.
- Role: Primarily involved in signaling the velocity and direction of muscle stretch, helping the CNS monitor the speed of movement.
- Secondary Spindle Receptors:
- Afferent Fibers: Innervated by Group II afferent fibers.
- Sensitivity: Less dynamic but more consistent in their response to static muscle length. They have a more regular discharge rate compared to primary receptors.
- Role: Provide information about static muscle length or limb position, aiding the CNS in maintaining posture and fine-tuning movements.
- Primary Spindle Receptors:
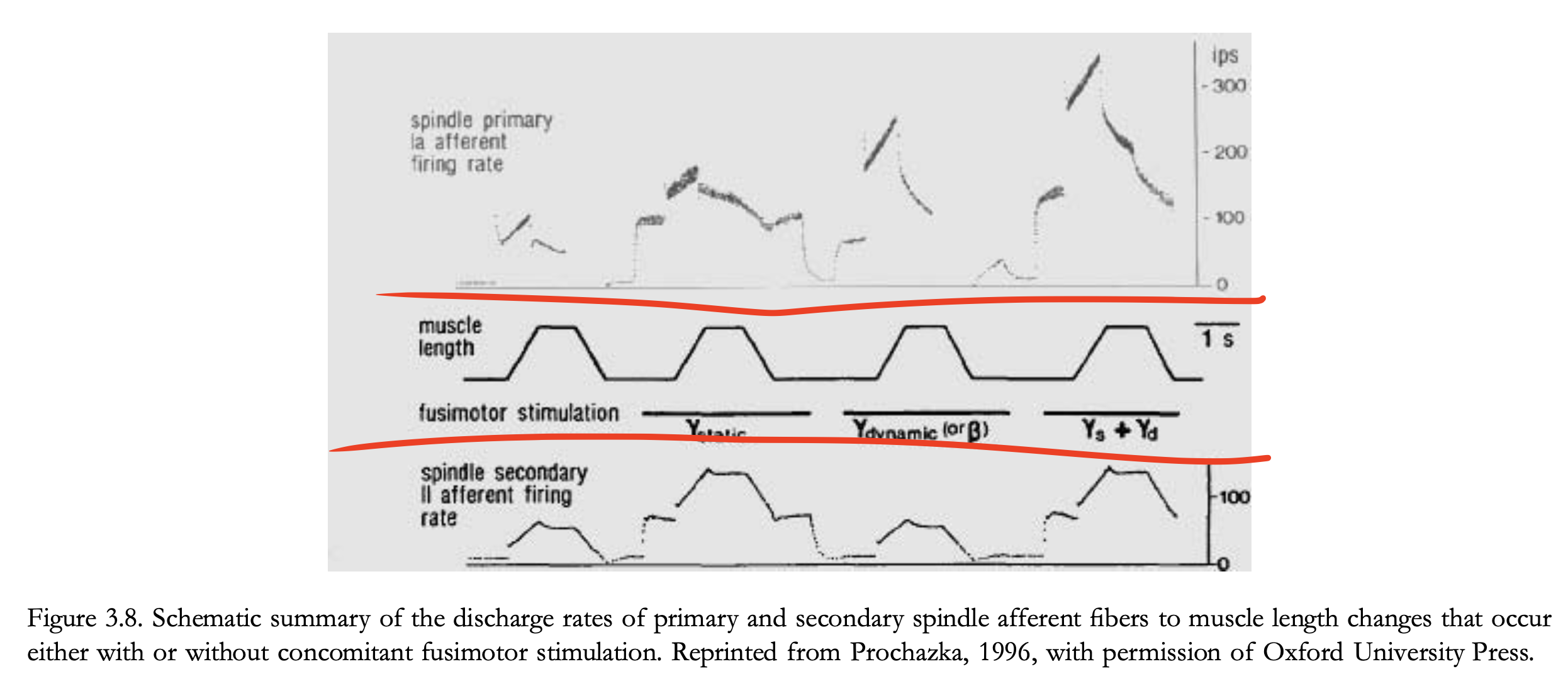
2. Golgi Tendon Organs
Location: Found at the junction between muscle tendons and extrafusal muscle fibers.
Function: These receptors respond to the force generated by muscle contractions, particularly the tension in the tendon.
Afferent Fibers: Innervated by Group Ib afferent fibers.
Role: They are sensitive to the tension generated by the muscle and provide the CNS with an overall measure of muscle force. This helps in preventing muscle damage from excessive force by triggering a reflex that inhibits further contraction.
Example: During heavy weightlifting, Golgi tendon organs help prevent muscle injury by signaling the CNS to reduce muscle force if it detects excessive tension.
Summary of Characteristics:
- Muscle Spindles Receptors: Detect muscle stretch and change in length, providing feedback on limb position and movement velocity.
- Golgi Tendon Organs: Detect muscle tension, preventing excessive force that could lead to injury.
These mechanoreceptors are essential for coordinated movement, balance, and the fine control of muscle activity. They enable the body to perform complex tasks, from lifting heavy objects to maintaining a stable posture, by continuously informing the brain about the state of muscles and joints.
What are the mechanorecptors in joins? What do they do?
Ruffini Endings, Golgi Endings, Paciniform Endings, Free Nerve Endings
Joint mechanoreceptors are specialized sensory receptors located in and around joint capsules. They play a crucial role in providing the central nervous system (CNS) with information about joint position, movement, and mechanical stress. Here are the main types of mechanoreceptors found in joints and their functions:
1. Ruffini Endings
- Characteristics: Slowly adapting receptors.
- Location: Found in joint capsules and ligaments.
- Function: Ruffini endings are sensitive to the stretching of joint capsules and provide information about the static position of the joint, as well as the direction and amplitude of joint movements.
- Example: During the gradual bending or stretching of a joint, Ruffini endings help the brain understand the extent of the movement and maintain a sense of joint position.
2. Golgi Endings
- Characteristics: Slowly adapting receptors, similar to those found in tendons (Golgi tendon organs).
- Location: Typically found in ligaments around joints.
- Function: These receptors respond to tension or stretch in the ligaments, especially at the extremes of joint motion (e.g., deep flexion or extension). They provide feedback on joint stress and may help protect the joint from injury by signaling when the joint is near its movement limits.
- Example: When the knee is bent to its maximum extent, Golgi endings in the knee ligaments signal the CNS to prevent overstretching and potential ligament damage.
3. Paciniform Endings
- Characteristics: Rapidly adapting receptors.
- Location: Found in joint capsules and periarticular connective tissue.
- Function: Paciniform endings are sensitive to rapid changes in joint position, particularly during the beginning and end of movement. They quickly adapt to sustained stimuli, which makes them ideal for detecting the initiation and cessation of movement rather than continuous joint position.
- Example: When you quickly rotate your wrist, Paciniform endings rapidly detect this motion and inform the brain of the dynamic change in position.
4. Free Nerve Endings
- Characteristics: Can be either slowly or rapidly adapting, often unmyelinated or lightly myelinated.
- Location: Distributed throughout joint capsules and surrounding tissues.
- Function: Free nerve endings are sensitive to mechanical stress, pain, and inflammation. They are responsible for detecting potentially harmful stimuli that could damage the joint, such as excessive stretching, and contribute to the sensation of pain.
- Example: If you twist your ankle, free nerve endings signal pain and alert the CNS to the potential injury, prompting a protective response.
Functional Role of Joint Mechanoreceptors
Distribution: The distribution of these receptors is non-uniform within the joint, reflecting the varying mechanical stresses experienced during movement. For example, certain receptors may be concentrated in areas that experience the most stretch or compression during joint motion.
Contribution to Proprioception: Joint receptors, particularly those active at the extremes of motion, complement the proprioceptive information provided by muscle spindles and Golgi tendon organs. While joint receptors provide some kinematic information during midrange motion, their role becomes more critical when muscle receptors are unable to contribute, such as when muscles are disengaged.
Redundancy in Sensory Feedback: Studies have shown that even in the absence of joint receptors, as seen in patients with joint prosthetics, other sensory systems (like muscle and skin receptors) can adequately compensate, ensuring that proprioception remains functional
In summary, joint mechanoreceptors are essential for monitoring joint position, movement, and stress. They work alongside muscle and skin receptors to provide comprehensive feedback to the CNS, helping to maintain joint stability and protect against injury.
Why does Corollay Discharges matter in the context of mechanorecptors?
Forcce sensing. 실제 peripheral sensing보다 Corollary Discharge로 force sensing을 더 함. 그리고 mechanoreceptors' discharge는 실제 힘 준거 + 외부 자극을 반영하기 때문에 실제 힘 준 거를 인식하기 위해 CD (EC)를 써야함.
The central nervous system appears to monitor its own activity in producing muscle contractions, and these correlates or copies of the motor command have been referred to historically as sensations of innervation (Helmholtz, 1866/1925) and more recently as corollary discharges or efference copies (McCloskey, 1981). The results from a number of experiments suggest that the perception of force is derived centrally from these internal neural correlates of the motor command and is not based on the activity of peripheral receptors in the muscle (Gandevia, 1996). Evidence to support this proposition comes from experiments in which it has been shown that as the motor command sent to a muscle increases, the perceived magnitude of the force of contraction also increases, even when the force exerted by the muscle remains constant (Jones & Hunter, 1983). This should not be interpreted as dismissing a role for peripheral receptors, such as Golgi tendon organs, in the perception of force as peripheral afferent activity is still required to indicate that the muscle force generated in response to the motor command is adequate to perform the task. A further role for corollary discharges is in the decoding of muscle spindle responses. The discharge rates of muscle spindle receptors are not only a function of changes in the length of the muscle but also reflect the activity of the fusimotorsystem.The central nervous system therefore needs to distinguish the spindle responses that are a consequence of fusimotor activity from those that are a result of an external perturbation and that have proprioceptive significance.
How does Sensory Cotrical Projections work?
In summary, sensory information from the hand and fingers is processed through complex pathways involving spinal cord and brain structures, resulting in a detailed and adaptive representation of sensory inputs in the cortex.
Sensory Pathways and Receptors
- Afferent Nerve Fibers and Dorsal Root Ganglion Neurons:
- Function: Sensory information from the hand's receptors and finger muscles travels through afferent nerve fibers to the dorsal root ganglion neurons. Each neuron has two branches: one extending to the periphery (skin and muscles) and the other to the central nervous system (CNS).
- Dermatomes: The area of skin served by a single dorsal root is called a dermatome. This concept is used to identify and diagnose spinal cord injuries by mapping skin responses to different stimuli.
- Different Sensory Modalities:
- Touch and Proprioception: Large-diameter axons involved in touch and proprioception (body position awareness) ascend through the dorsal columns of the spinal cord. These axons are divided into two fascicles: the gracile fascicle (medial, from lower body) and the cuneate fascicle (lateral, from upper body). They terminate in the gracile and cuneate nuclei in the medulla, then cross to the opposite side and ascend to the thalamus through the medial lemniscus.
- Temperature and Pain: Smaller-diameter axons that carry information about temperature and pain terminate in the dorsal horn of the spinal cord and form the anterolateral system. These axons cross the midline and ascend through the spinal cord to the thalamus, with projections to various cortical areas.
Cortical Processing:
- Primary Somatosensory Cortex (S1):
- Areas: The sensory information reaches different areas of the primary somatosensory cortex (S1) in the parietal lobe: areas 3a and 3b (proprioception and touch), and areas 1 and 2 (further processing of tactile and proprioceptive information).
- Motor Coordination: Area 2 sends significant projections to the motor cortex, impacting hand function and coordination. Damage to this area can impair finger movements even without affecting muscle strength.
- Cortical Representation and Plasticity:
- Body Mapping: The S1 contains a map of the body where areas with higher sensory innervation (e.g., fingertips) have larger cortical representations. This map is dynamic and can change based on sensory experience.
- Training and Deafferentation: Training or extensive use of specific body parts (e.g., playing a musical instrument) can enlarge the cortical representation of those parts. Conversely, loss of sensory input (e.g., amputation) can cause adjacent areas of the cortex to expand into the deafferented region.
- Illusions of Size:
- Anesthesia Effects: Local anesthesia can cause an illusory increase in the perceived size of the anesthetized body part (e.g., thumb or lips). This effect may result from changes in cortical representations and the unmasking of sensory inputs adjacent to the anesthetized area.
What are special about Motor Cortical Areas?
- Specialization and Overlap: Each motor cortical area has specialized functions but works in concert with others. For example, M1 is directly involved in motor execution, while SMA and pre-SMA are more focused on planning and sequencing.
- Dynamic Organization: The motor cortex shows remarkable plasticity. Its organization can adapt based on experience and skill development, such as through motor learning or following injury.
- Integration of Sensory and Motor Information: Areas like the PM integrate sensory information to guide and refine motor actions, highlighting the importance of sensory feedback in motor planning and execution.
Motor Cortical Areas and Their Roles
- Primary Motor Cortex (M1 or Area 4):
- Role: Directly controls voluntary movements, particularly of the distal muscles of the contralateral arm. It is involved in executing specific motor commands and has multiple representations for each muscle.
- Key Takeaway: M1 contains detailed and overlapping maps for different fingers and hand muscles, with a large area dedicated to hand control. Stimulation of different points within M1 can activate the same muscle, indicating a complex and redundant organization for precise motor control.
- Supplementary Motor Area (SMA or Medial Part of Area 6):
- Role: Involved in planning and coordinating sequential movements and arranging multiple movements in a proper temporal order. It plays a role in complex movement sequences.
- Key Takeaway: SMA is active during the performance of sequential movements and helps in organizing and planning these movements, showing its importance in executing complex motor tasks.
- Presupplementary Motor Area (pre-SMA or Part of Area 6):
- Role: Functions in preparing and updating information for upcoming sequences of movements. It is particularly active during the preparation phase before a movement.
- Key Takeaway: Pre-SMA is critical for the cognitive aspects of motor planning, particularly for preparing and organizing the sequence of movements before they occur.
- Premotor Cortex (PM or Lateral Part of Area 6):
- Role: Involved in the planning and execution of movements guided by sensory information. The ventral PM participates in visual guidance of movements, while the dorsal PM is associated with movement planning and rehearsal.
- Key Takeaway: The PM is important for integrating sensory inputs with motor actions, with different regions specialized for visual guidance and movement planning.
Additional Insights
- Corticospinal Tract: The primary motor cortex and other motor areas project to the spinal cord via the corticospinal tract. About 40% of the fibers in this tract originate from M1, highlighting its crucial role in direct motor control.
- Motor Skill Learning and Cortical Plasticity: Motor learning induces changes in the cortical representation of hand movements. The motor cortex's representation can expand with practice and shrink in response to deafferentation or injury, reflecting its adaptability.
- Somatotopic Organization: The organization of motor cortex areas is dynamic and can change with motor experience or injury. For example, after amputation, the cortical area previously dedicated to the missing limb may be reassigned to adjacent muscles.
'Research (연구 관련)' 카테고리의 다른 글
Attributes of Wielded Objects (0) | 2024.08.19 |
---|---|
HHF Part4 - Tactile Sensing (0) | 2024.08.14 |
HHF Part2 - Evolutionary Development and Anatomy of the Hand (0) | 2024.08.05 |
HHF Part1 - Historical Overview and General Introduction (0) | 2024.08.05 |
Pytorch Lightning (0) | 2024.08.02 |
- Total
- Today
- Yesterday
- 문경식
- part segmentation
- Generative model
- VAE
- Docker
- 피트니스
- 에디톨로지
- densepose
- pytorch
- Machine Learning
- Virtual Camera
- 비전
- focal length
- 헬스
- 컴퓨터비전
- spin
- 2d pose
- 컴퓨터비젼
- nohup
- Interview
- pyrender
- demo
- 머신러닝
- Transformation
- 인터뷰
- Pose2Mesh
- world coordinate
- nerf
- camera coordinate
- deep learning
일 | 월 | 화 | 수 | 목 | 금 | 토 |
---|---|---|---|---|---|---|
1 | 2 | 3 | ||||
4 | 5 | 6 | 7 | 8 | 9 | 10 |
11 | 12 | 13 | 14 | 15 | 16 | 17 |
18 | 19 | 20 | 21 | 22 | 23 | 24 |
25 | 26 | 27 | 28 | 29 | 30 | 31 |