티스토리 뷰
Part 10: Applied Aspects of Hand Function
evaluation of hand function and rehabilitation, language communication with the hand, sensory communication systems for the blind, haptic interfaces, and exploring art by touch
8/26/2024
"Evaluation of Hand Function and Rehabilitation"
Muscle and Joint Function
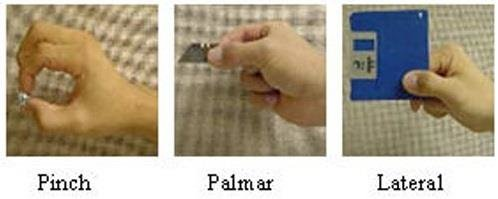
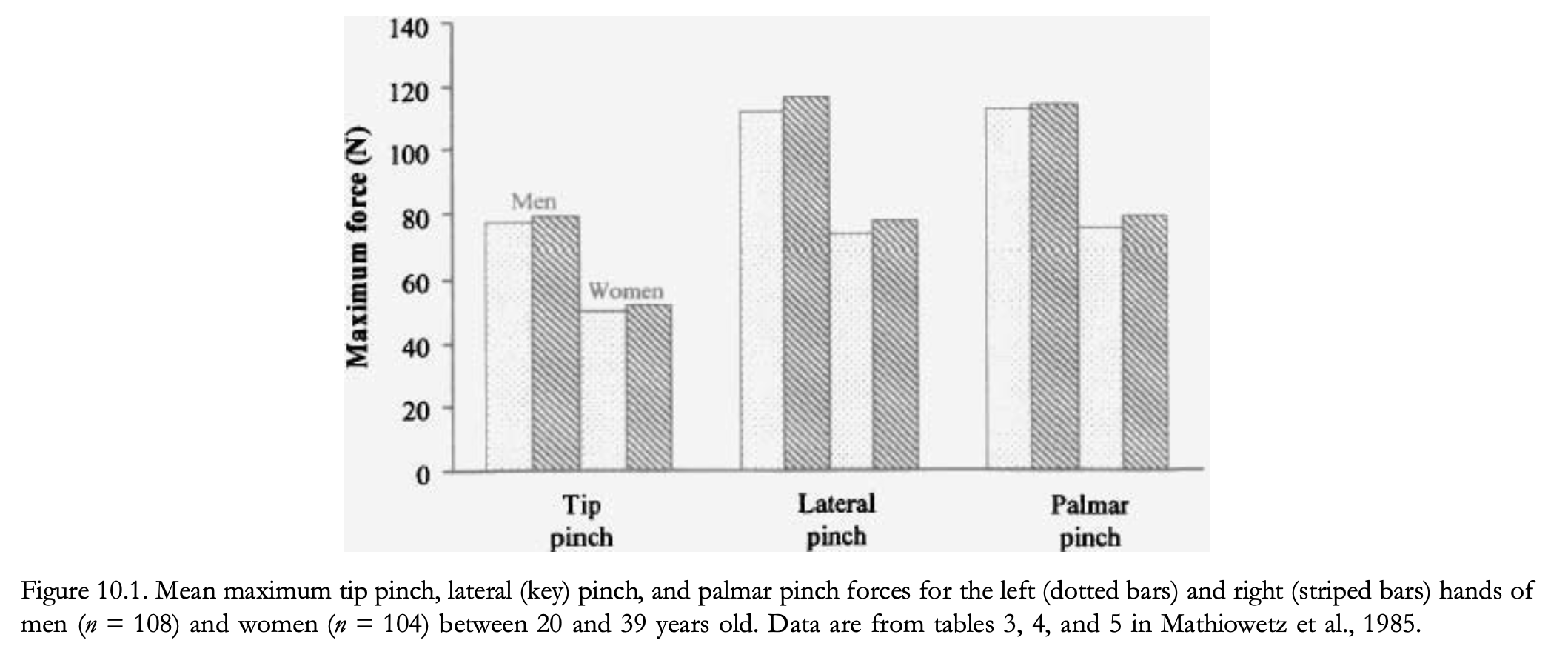
- Grasp and Task Influence on Muscle Force: The force that muscles controlling the hand can generate depends on the type of grasp used, which varies based on the task being performed.
- Grip Strength and Health: Grip strength is an important indicator of neurological and musculoskeletal health. A loss in grip strength is linked to various conditions, making it a common test in hand evaluations.
- Normative Data for Grip Strength: Extensive studies have established normative data on grip strength for different populations, highlighting the importance of considering factors like sex, age, and hand preference when interpreting results.
- Sex, Age, and Grip Strength:
- Women typically have about 60% of the grip strength of men.
- Grip strength peaks in the third decade of life and declines with age.
- Differences in grip strength between hands should be cautiously interpreted, as some individuals may have equal or greater strength in their non-preferred hand.
- Precision Grips:
- The maximum forces produced with precision grips (tip, palmar, and lateral pinch) are important in clinical assessments.
- Palmar and lateral pinches generate about 40% more force than tip pinches.
- Age and sex influence the maximum forces generated in these grips, similar to grip strength.
- Grip Force for Daily Activities:
- A grip force of approximately 40N is sufficient for most daily activities.
- A pinch force of 10N is adequate for most simple grasping tasks.
- Range of Motion Assessments:
- The passive and active ranges of motion in hand joints are measured using a goniometer.
- Guidelines exist for the starting position and goniometer placement during these assessments.
- The data can be compared to normative values to assess the severity of impairments.
Sensory Testing
- Tactile Sensibility Tests:
- Common Tests: Tactile sensibility of the hand is evaluated using tests like two-point discrimination, pressure sensitivity, and haptic object recognition.
- Specialized Tests: Vibratory threshold testing and thermal sensitivity tests are used for specific conditions, such as peripheral nerve injuries.
- Vibratory Testing Limitations:
- Vibratory testing is less informative for individuals with cortical lesions, as they may retain vibration perception despite deficits in other tactile functions.
- Two-Point Discrimination:
- Frequently used to assess spatial acuity, especially on the palmar surfaces of the distal phalanges.
- The accuracy of this test can be influenced by factors like the force applied and examiner variability.
- Some researchers argue that it may not be a true measure of tactile spatial acuity.
- Alternative Measures for Spatial Acuity:
- Tests like gap detection and grating orientation are promising for assessing fine spatial detail and are less influenced by non-spatial cues.
- Dynamic Two-Point Discrimination:
- A variation that involves moving the stimulus along the finger, often used to assess sensory recovery after nerve injuries.
- Dynamic thresholds are more sensitive and can show better recovery compared to static thresholds.
- Tactile Localization:
- Important for assessing sensory function, especially in individuals with peripheral nerve injuries or cortical lesions.
- Mislocalization of tactile stimuli can occur due to disrupted spatial projections to the cortex or cortical reorganization.
- Pressure Sensitivity:
- Evaluated using Semmes-Weinstein monofilaments and compared to normative data.
- Correlated with two-point discrimination thresholds but measures different aspects of tactile sensation.
- Haptic Object Recognition:
- A critical component of neurological exams, involving the ability to identify objects by touch.
- Deficits in haptic recognition are associated with peripheral nerve damage or cortical lesions, particularly in the parietal lobe.
- Manual Exploratory Procedures:
- The strategies used to explore objects haptically can influence the quality of sensory information obtained.
- Studying these strategies in individuals with sensory loss could inform sensory rehabilitation techniques.
- Proprioceptive System Evaluation:
- Commonly assessed in individuals with cortical or subcortical lesions through tests like passive movement detection and weight discrimination.
- Weight discrimination is an active process and may provide a better indicator of proprioceptive system status.
Functional Tests
- Hand Function vs. Sensory Tests: Sensory tests don't reliably predict hand function, leading to the development of function-focused tests like Moberg's picking-up test.
- Moberg’s Test: The picking-up test, where subjects pick up objects blindfolded, correlates with two-point discrimination but not other sensory tests.
- Functional Test Development: Moberg’s test inspired other hand function tests, which usually measure performance by time or response count.
- Limitations: These functional tests often ignore movement dynamics and force, focusing on basic performance metrics instead.
"Language Communication with the Hand"
- Versatility of the Hand in Communication: The hand is a vital tool for communication, especially for individuals with disabilities. It can convey information through various methods, such as sign language, Braille, and tactile methods like Tadoma.
- Tadoma Method: Tadoma is a tactile method where a person places their hand on a speaker's face to feel the movements and vibrations associated with speech. This method, developed in the late 19th century, allows individuals who are both deaf and blind to understand speech through tactile feedback.
- Effectiveness of Tadoma: Tadoma has been shown to be as effective as listening to speech in noisy environments for individuals who are trained in its use. It also outperforms artificial tactile displays of speech in conveying linguistic information.
- Haptic Reception of Sign Language: For deaf-blind individuals, haptic reception involves placing their hands on the signer's hands to perceive sign language. Although this method is slower and more error-prone than visual sign language, it remains an effective communication tool.
- Information Transfer Rates: The passage compares various communication methods in terms of information transfer rates. Spoken language and visual sign language have the highest rates, while methods involving sequential letter presentations (like Morse code or finger spelling) are less efficient but can still be accurate.
- Comparison with Speech: While hand-based communication methods generally have lower output rates compared to speech, signing and typing on a stenography machine can approach the efficiency of spoken communication.
09/01/2024
"Sensory Communication Systems for the Blind"
Tangible Graphics
- Inferior Spatial Acuity: The fingertips have lower spatial resolution compared to the visual system's fovea. This means the haptic system struggles with processing fine spatial details.
- Slow and Inaccurate Contour Following: When using static raised-line displays, the haptic system relies on a method called "contour following" to understand two-dimensional shapes. This process is slow and often inaccurate, making it difficult to form an overall spatial representation.
- Heavy Memory Load: The sequential nature of haptic exploration imposes a significant memory burden on users. This makes it hard to integrate the information into a coherent spatial layout.
- Difficulty in Grouping Spatial Information: Users often find it challenging to parse and group the two-dimensional information provided by raised-line displays, leading to confusion.
- Limited Effectiveness of Edge-Based Information: Unlike the visual system, which can effectively use edges to recognize objects and perceive spatial layouts, the haptic system is less skilled in doing so.
- Coarse Haptic Information: Even when using the whole hand for exploration, the haptic system provides spatially coarse information compared to the detailed spatial information available through vision.
- Poor Performance in Object Recognition: Raised-line representations of common objects are often poorly recognized through haptic exploration, especially when compared to direct object manipulation.
Suggested Improvements
- Adjustment of Two-Dimensional Details: Due to the imprecision of the haptic system in processing fine geometric details, map designers suggest omitting unnecessary details, enlarging important ones, and potentially distorting features to improve comprehension on tactile maps.
- Enhancing Three-Dimensional Structure: Introducing three-dimensional variations in tactile displays can improve recognition and comprehension, as the haptic system is better at processing three-dimensional geometric cues through grasping.
- Haptic Interfaces: Advanced haptic interfaces, which provide force feedback, offer the potential to create virtual three-dimensional displays that may enhance the identification of objects and their spatial layouts compared to static raised-line displays.
- Material Cues: Adding material variations (like texture or compliance) to tactile displays can enhance their effectiveness, as the haptic system is particularly adept at processing material differences.
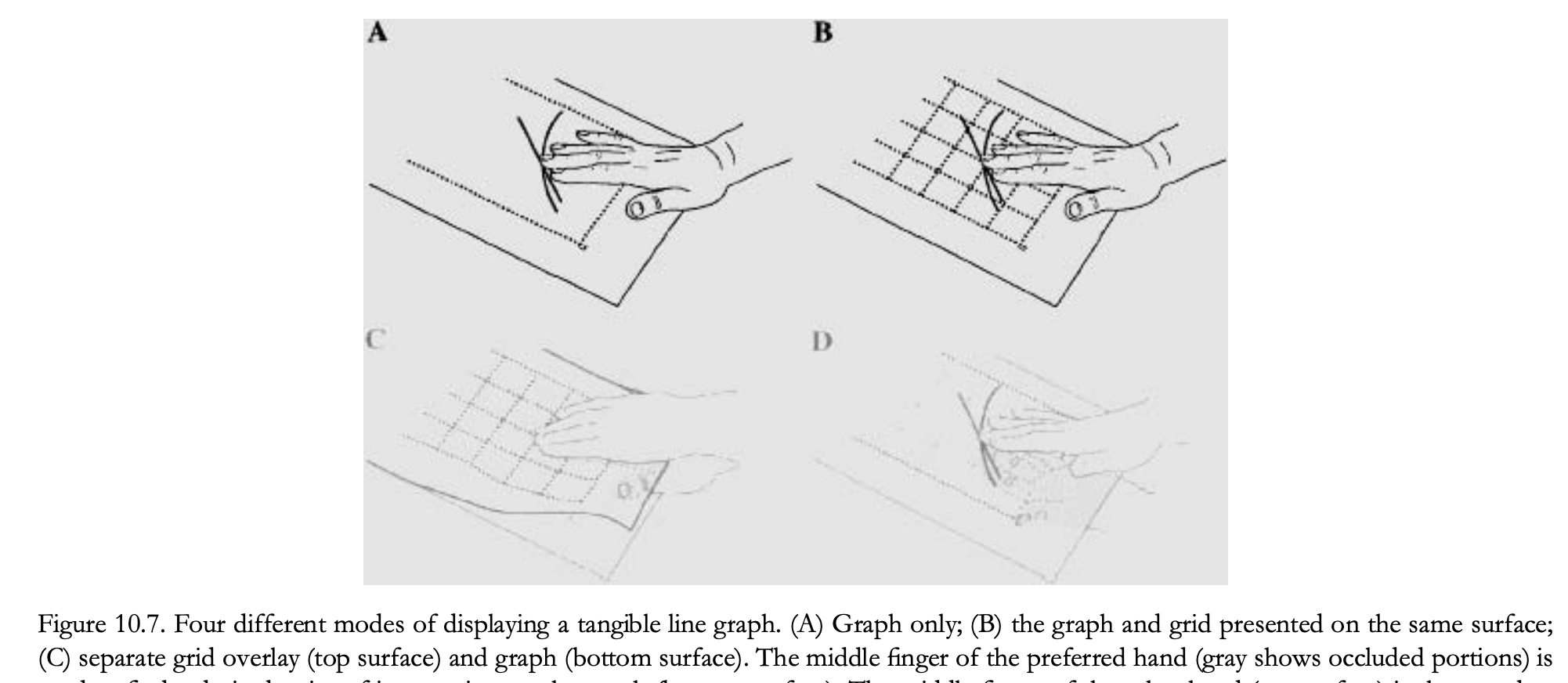
- Reducing Tactile "Noise": To avoid clutter in tactile displays, reducing unnecessary symbols and using techniques like underlays to separate important information from background data can help users process spatial information more effectively. -> 좀 흥미로움
- Choosing Display Symbols: The selection of tactile symbols must consider their tangibility and meaningfulness. Symbols should be large enough to be distinguishable but not so large that they create clutter, and their physical structure should ideally reflect their meaning to reduce identification time.
- Symbol Standardization: While standardizing tactile symbols could be beneficial, achieving complete standardization is challenging due to the limited number of differentiable tactile symbols and the need for them to be effective in various contexts.
- Figure-Ground Organization: Understanding how the haptic system organizes spatial information and separates figure from ground is crucial for designing effective tactile displays, but research in this area is still limited.
- Multisensory Cues: Integrating additional sensory cues, particularly auditory, into tactile displays can improve performance. Multimodal displays that combine haptic and auditory information have shown promising results, especially in simpler graphic formats like bar charts. -> 좀 흥미로움
Production Methods:
- Impact of Production Method: The effectiveness of tangible graphic displays is highly dependent on the chosen production method, with traditional methods being labor-intensive and difficult to modify.
- Traditional Methods: Early methods, like manually arranging materials or using metal foil, were time-consuming but allowed for effective tactile cues.
- Swellpaper Technology: Introduced in the 1970s, swellpaper became popular for its ease of use in creating raised graphics, despite lacking precise height control.
- Vacuum-Forming and Electromechanical Systems: Vacuum-forming is less precise than swellpaper, while electromechanical systems are costly and less reliable, limiting their accessibility.
- Digital and Vibrotactile Solutions: Digital solutions, like the VirTouch Mouse, offer new ways to interact with tactile displays, though improvements in performance are modest.
- 3D Rapid Prototyping: Technologies like stereolithography offer accurate, efficient methods for creating three-dimensional tactile displays.
System for Denominating Banknotes by Touch:
Challenges for the Blind: Identifying banknotes is difficult for blind individuals, leading to various global approaches, but no universal solution.
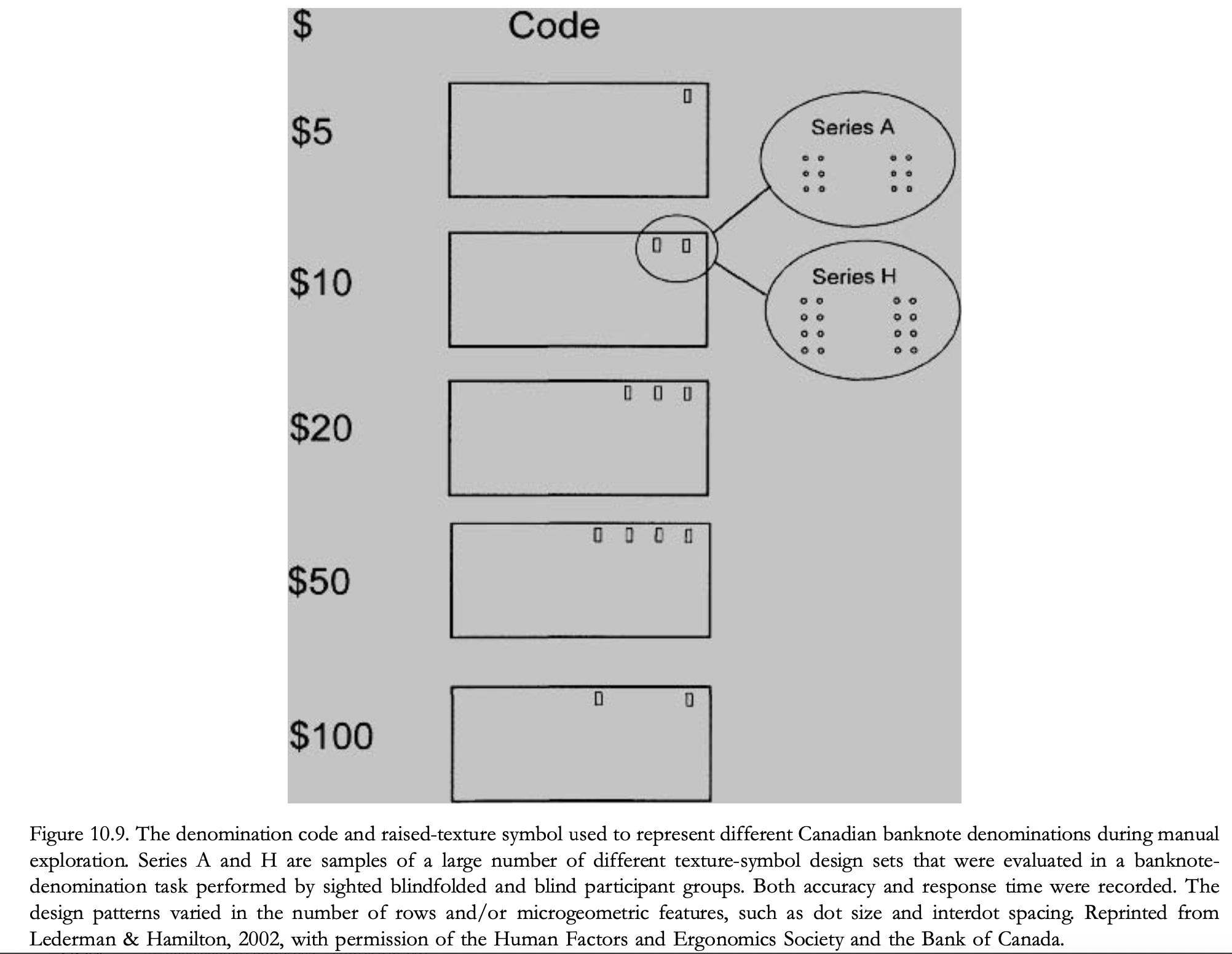
Canadian Tactile Code: The new Canadian banknote series uses a tactile code with raised texture patches to represent denominations, designed for easy and accurate identification.
"Haptic Interfaces"
- Definition and Function: Haptic interfaces provide tactile and force feedback to users interacting with real or simulated environments via a computer, allowing them to feel and manipulate virtual objects.
- Design Considerations: Effective haptic interfaces require a deep understanding of the human haptic system, including sensitivity to force, vibration, and rigidity, which has driven interdisciplinary research and development.
- Advancements: Haptic interfaces have evolved from simple devices like joysticks to more sophisticated systems that provide detailed tactile information to the fingertips or whole hand, enhancing realism and task performance in various applications.
- Applications: Haptic feedback is critical in environments where visual information is limited, such as underwater, in space, or inside the human body. It improves performance in tasks requiring manipulation by providing feedback on properties like mass, texture, and rigidity.
- Emerging Uses: Haptic interfaces are increasingly used to manage visual overload in critical environments like air traffic control by providing alternative sensory alerts, and in scientific visualization to enhance understanding of complex data.
- Types of Haptic Devices: The term "haptic device" includes both tactile and force-feedback displays, which differ in the feedback they provide. Force-feedback devices resist user movements, while tactile-feedback devices offer information about surface textures and contact without resisting motion.
- Device Configurations: Haptic devices vary widely in form, including gloves, exoskeletons, and styluses. Research on optimizing these designs for specific tasks and user comfort is still relatively limited.
- Force vs. Tactile Feedback: Force-feedback systems convey information about mechanical properties like weight and inertia, actively resisting motion, while tactile-feedback systems provide surface and texture information without resistance.
Force-feedback devices differ from tactile-feedback devices in that they are usually larger and heavier due to the force output requirements of the actuators. These devices may be attached to the hand and arm in the form of an exoskeleton or mounted on the floor or a desk, such as a joystick. The latter interfaces are termed earth-grounded, off-the-body systems and typically have fewer degrees of freedom than do exoskeletal devices. Several force-feedback devices are illustrated in figure 10.10.
Difference Between Tactile-Feedback and Force-Feedback Interfaces:
- Type of Feedback:
- Tactile-Feedback Interfaces: Provide surface-level information like texture, pressure, and vibration. They stimulate the skin, usually through small actuators, pin arrays, or vibrators. These devices give feedback about what is felt on the surface of an object but do not resist the user's movements.
- Example: The iFeel Mouse provides subtle vibrations when interacting with computer icons, and the CyberTouch glove uses vibrotactile actuators on the fingers to simulate contact with virtual objects.
- Force-Feedback Interfaces: Provide kinesthetic feedback by simulating resistance, weight, or force, allowing users to feel the mechanical properties of objects like stiffness, inertia, or friction. These devices can actively resist or guide user movements.
- Example: The PHANTOM device allows users to feel the weight and resistance of virtual objects, while the CyberGrasp glove uses actuators to simulate the force needed to grasp objects, enhancing the sense of manipulating a real object.
- Tactile-Feedback Interfaces: Provide surface-level information like texture, pressure, and vibration. They stimulate the skin, usually through small actuators, pin arrays, or vibrators. These devices give feedback about what is felt on the surface of an object but do not resist the user's movements.
- Physical Characteristics:
- Tactile-Feedback Interfaces: Typically lighter and smaller, designed to be worn or held, and focus on delivering precise but less forceful feedback.
- Example: The CyberTouch glove offers vibratory feedback at the fingertips, which is useful in virtual environments where detailed touch information is required.
- Force-Feedback Interfaces: Often larger and more robust, capable of producing significant forces, and can be mounted or worn as an exoskeleton. They provide a more immersive and physically engaging experience by simulating the feel of real-world interactions.
- Example: The CyberGrasp glove uses a mechanical exoskeleton to provide substantial resistance to finger movements, simulating the act of gripping and manipulating objects with realistic force feedback.
- Tactile-Feedback Interfaces: Typically lighter and smaller, designed to be worn or held, and focus on delivering precise but less forceful feedback.
In summary, tactile-feedback interfaces focus on surface sensations like texture and vibration, while force-feedback interfaces simulate the physical interaction by providing resistance and force, allowing users to feel weight and resistance.
Explore Art by Touch
Museums like San Francisco's Exploratorium and projects like the EU's PURE-FORM are exploring ways to incorporate tactile feedback in art and virtual environments. Visual artist Rosalyn Driscoll creates sculptures designed to be explored by touch, challenging the notion that the blind can't appreciate art. The importance of active hand movements in haptic perception is emphasized, with implications for the design of robotic systems in teleoperation and rehabilitation.
'Research (연구 관련)' 카테고리의 다른 글
Capture data from ARIA glasses (0) | 2024.08.30 |
---|---|
SSH from Remote (0) | 2024.08.30 |
Model Parallelisum (0) | 2024.08.23 |
HHF Part9 - Hand Function Across the Lifespan (0) | 2024.08.23 |
HHF Part8 - End-effector Constraints (0) | 2024.08.21 |
- Total
- Today
- Yesterday
- 에디톨로지
- pyrender
- nerf
- Virtual Camera
- pytorch
- focal length
- world coordinate
- 헬스
- 머신러닝
- Docker
- 비전
- part segmentation
- 컴퓨터비젼
- camera coordinate
- Transformation
- 피트니스
- 문경식
- demo
- 인터뷰
- Generative model
- 컴퓨터비전
- nohup
- Machine Learning
- 2d pose
- deep learning
- densepose
- Pose2Mesh
- Interview
- spin
- VAE
일 | 월 | 화 | 수 | 목 | 금 | 토 |
---|---|---|---|---|---|---|
1 | 2 | 3 | ||||
4 | 5 | 6 | 7 | 8 | 9 | 10 |
11 | 12 | 13 | 14 | 15 | 16 | 17 |
18 | 19 | 20 | 21 | 22 | 23 | 24 |
25 | 26 | 27 | 28 | 29 | 30 | 31 |